Hyperspectral cameras combine imaging and spectroscopy to measure light from a scene in hundreds of contiguous spectral bands. A two-dimensional image is created, with each pixel containing a full reflectance or transmission spectrum. Resulting data can be used to identify chemical composition, changes in material properties, or differentiate plant species (amongst many other applications). As an example, Figure 1 below shows hyperspectral imaging data of vegetation and a few representative spectra.
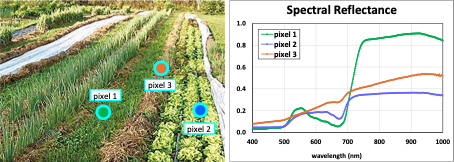
Attaching a hyperspectral camera to a microscope enables spectroscopic data from microscope images, providing high-resolution spectral data for an almost unlimited number of applications (for a recent and wide-ranging review of applications in research and industry, see reference [1]). A brief and incomplete, list of topics addressed with hyperspectral microscopy includes the following:
- All branches of biology
- Medicine and pathology
- Agriculture and soil science
- Food science and quality control
- Environmental sciences and monitoring
- Materials science
- Nanotechnology
- Textiles
- Forensics
- National security
Most standard commercial hyperspectral cameras use a line-scan (colloquially called “pushbroom”) approach to imaging, where each camera frame yields a one-dimensional line of pixels. A full two-dimensional image is constructed by translating the sample relative to the camera while simultaneously acquiring many camera frames, and stacking the lines of pixels next to each other.
Specialized equipment is needed for pushbroom imaging, depending on how and where the camera is used: in a laboratory, outside in the field, or flown from an aircraft. A typical laboratory system for scanning macroscopic objects, such as plants and food items, will mount the hyperspectral camera on a tower above lighting fixtures, while the subject sits on a linear translation stage or conveyor belt below the camera. During data acquisition, the subject moves while the camera remains stationary. See Figure 2.
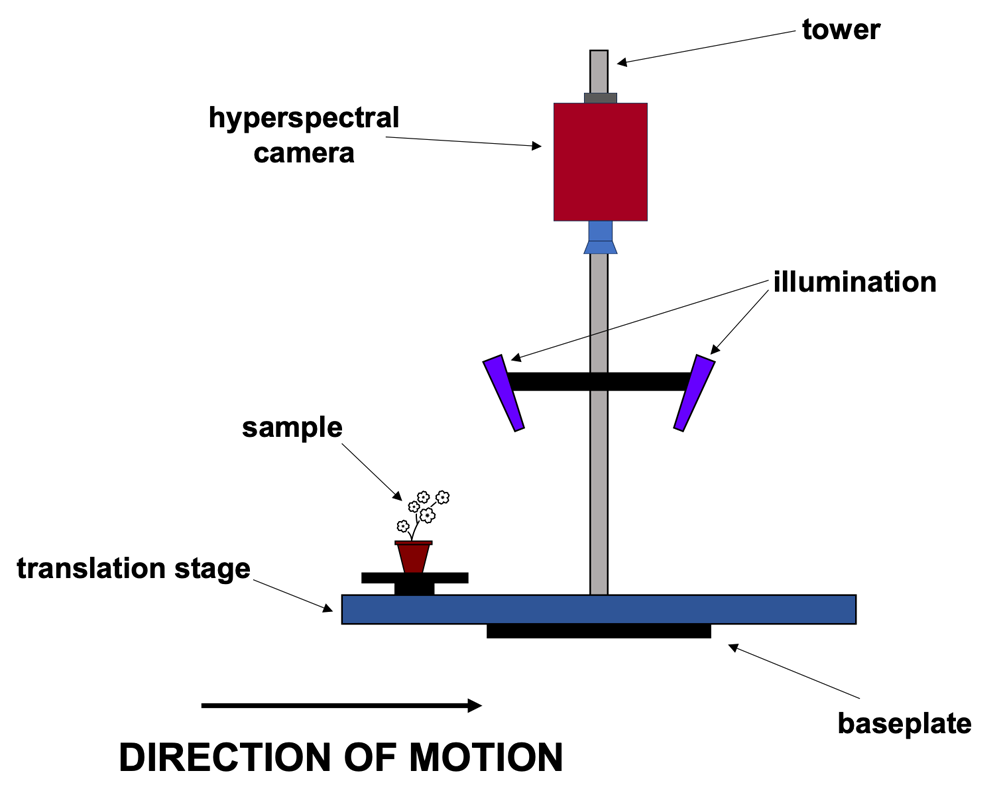
In outdoor field systems, the hyperspectral camera sits on a rotation stage on top of a tripod, and the hyperspectral camera rotates to scan the scene, as shown in Figure 3. In airborne remote sensing systems, the hyperspectral camera moves with the aerial vehicle, see Figure 4.
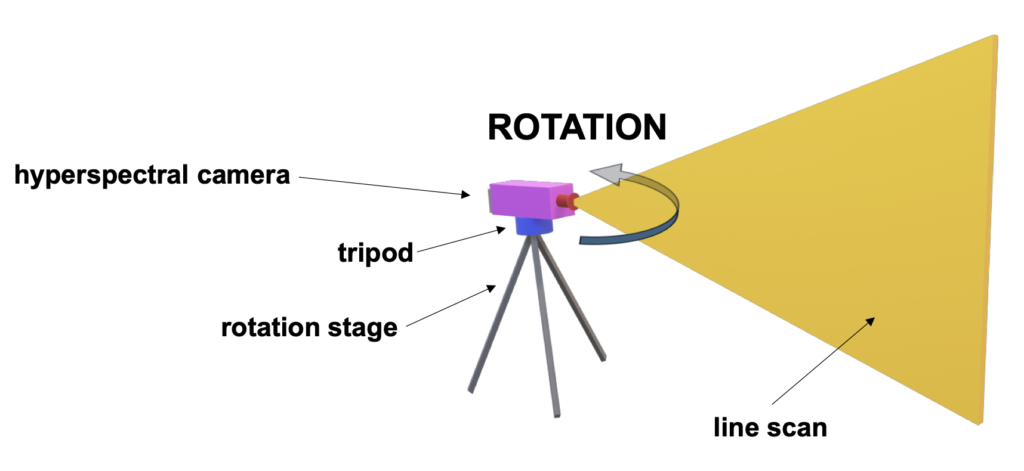
Acquiring high-quality images using pushbroom cameras requires precisely synchronizing camera frame rates with motion of the sample or camera. Commercial hyperspectral systems commonly come with proprietary software that accomplishes this task, being fully integrated with translation and rotation stages.
However, integration with motorized microscope stages is uncommon and, when available, quite expensive. Although many commercially available hyperspectral cameras can attach to the camera port of a microscope, without stage-software integration hyperspectral data cannot be obtained.
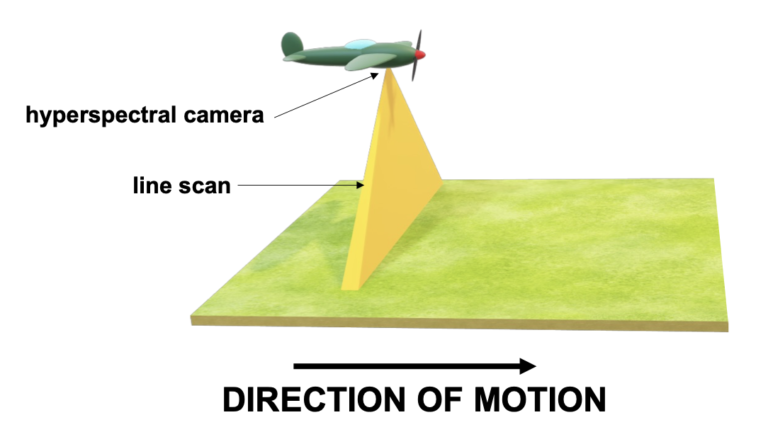
A method that circumvents this obstacle is Surface Optic’s unique internal scanning system. All moving parts in this design are contained within the hyperspectral camera, so that full two-dimensional scanning occurs from a stationary package. The case and objective lens are held stationary while the spectrograph and sensor, connected as a single unit, translate across the objective lens’ field of view. See Figure 5.
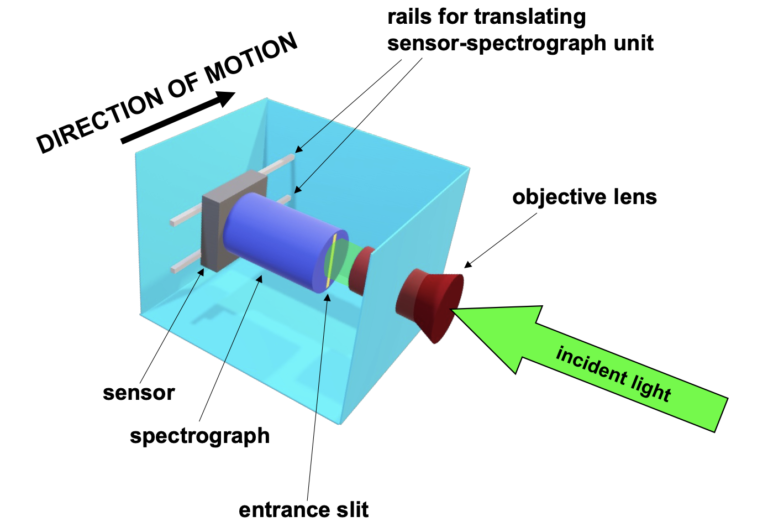
Surface Optics’ internal scanning system is ideal for use with microscopes, as no additional stage integration is required. The user simply installs the 710 into the camera port of a microscope and the resulting microscope image can be scanned.
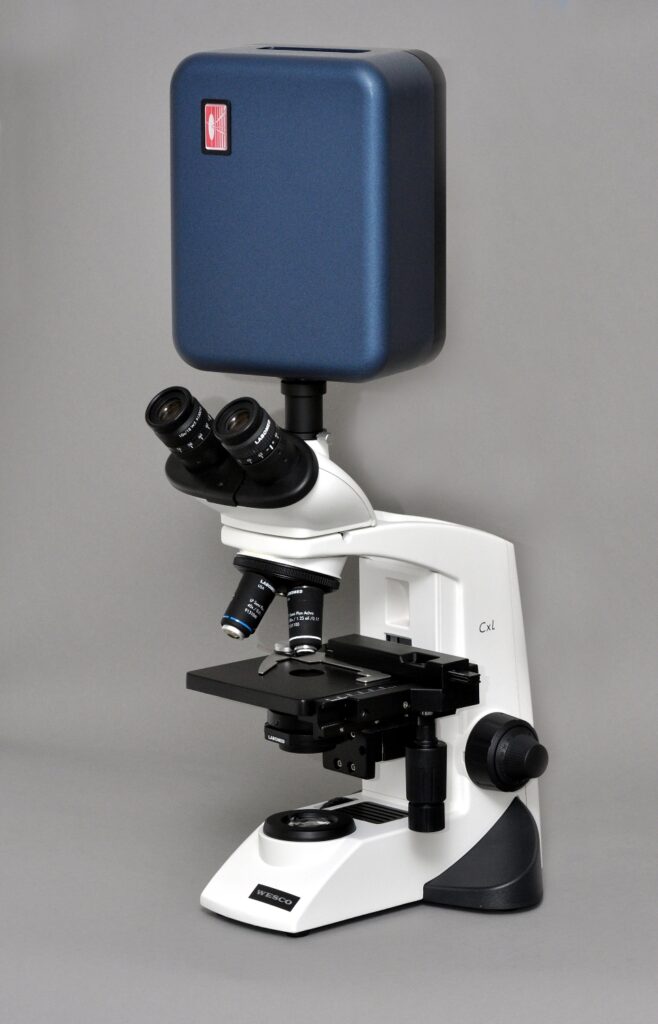
Surface Optics’ data acquisition software synchronizes camera frame rates and internal stage motion, and it is remarkably straightforward to generate hyperspectral images of microscopic subjects. Data is saved in standard binary formats and easily accessible by common software tools. In this example (Figure 7), the 710-VP is mounted on a microscope via a C-mount, utilizing the microscope objective in place of a traditional HSI lens. Data cubes were collected at 10x magnification from an aged leaf specimen between two glass slides, revealing distinct heterogeneity within the leaf structure.
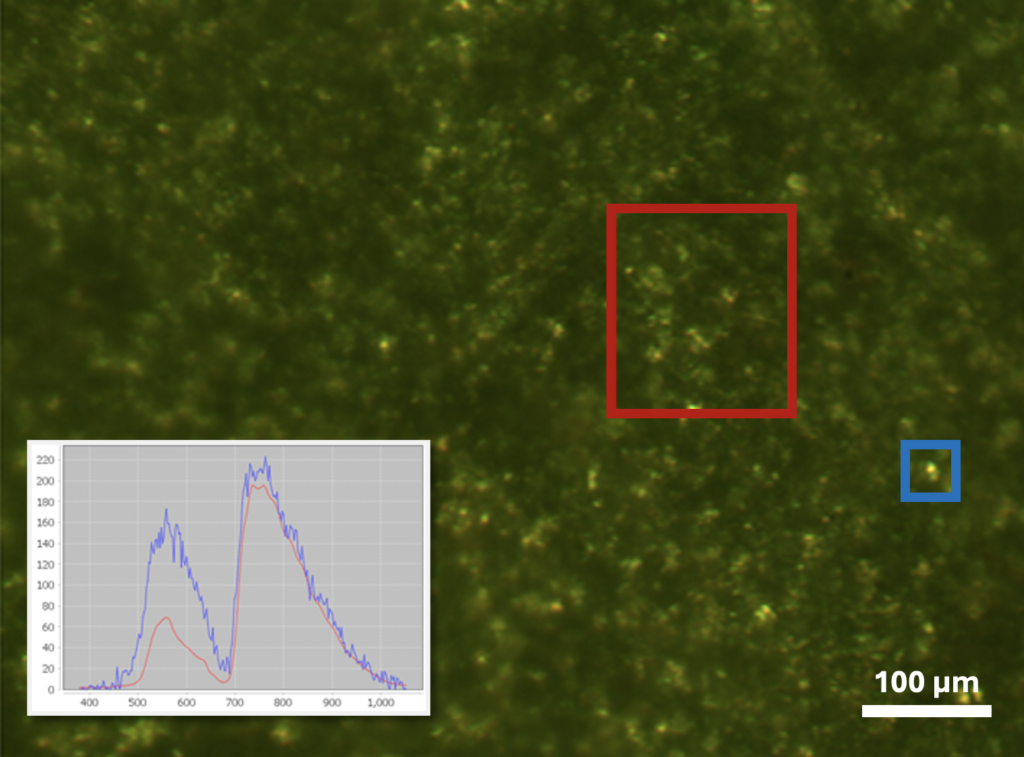
In this configuration, the leaf is back-illuminated, and the recorded spectra represent transmission rather than the typical reflectance. A bright area (highlighted in the blue box) indicates yellowing due to chlorophyll degradation, a sign of leaf aging. Interestingly, absorption in the red band remains high, possibly due to a more resilient form of chlorophyll or another background absorbing material. This image was collected without modification to our benchtop microscope or the 710-VP—the internal line scanning of the 710-VP does not required a motorized microscope stage.
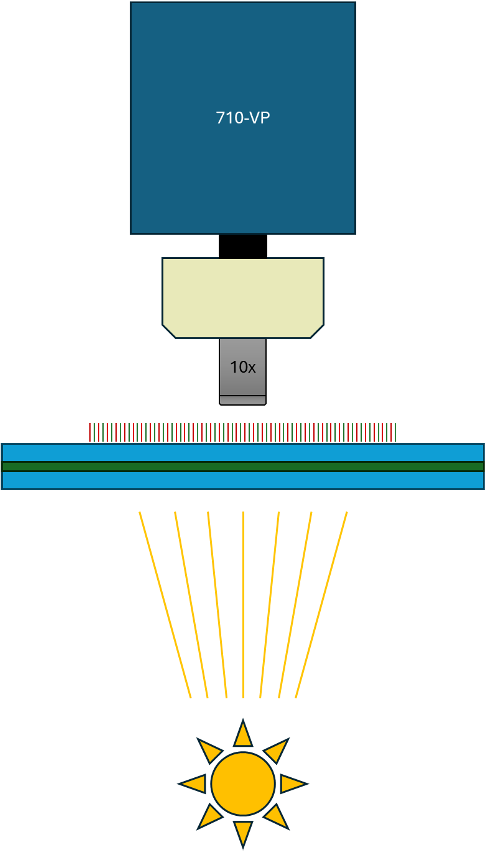
One alternative to pushbroom imaging, and well suited to hyperspectral microscopy, is snapshot hyperspectral imaging. These cameras generate a full 2-dimensional image in one camera frame but at the expense of lowered spatial and spectral resolutions. Unlike pushbroom imaging, because a snapshot image is created in a single frame, there is no need to translate the sample relative to the camera – it is unnecessary to integrate it with an external stage.
Snapshot hyperspectral imaging cameras typically use special sensors that have micro-filters attached to each sensor pixel. A single spatial pixel is constructed by combining data from many adjacent sensor pixels, each with a different filter. As a result, snapshot imagers have fewer spatial and spectral channels than pushbroom imagers. There can also be significant crosstalk between adjacent spectral channels, effectively raising the noise floor and lowering SNR. Despite these disadvantages, however, because snapshot imagers need only one frame to create a complete 2-dimensional hyperspectral image, they offer video-rate capabilities and potentially new fields of study.
Extending hyperspectral microscopy into the infrared (IR) wavelengths offers additional insights and will eventually provide valuable information in cellular, biomedical, and materials sciences. However, this experimental technique is not currently in widespread use, and its applications still need to be explored. The SOC-710-SWIR hyperspectral scanning system is an ideal instrument for obtaining IR hyperspectral data on microscopic subjects; it uses an InGaAs sensor to capture light with wavelengths in the 900 to 1700 nm spectral range, where many interesting spectral features occur. Future research in this field is destined to exciting and open new avenues for innovation.
Conclusion
Surface Optics’ unique internal scanning system is ideal for hyperspectral microscopy, avoiding difficult and expensive hardware-software synchronization between camera frame rates and microscope stage motion. Surface Optics’ SOC-710 line of hyperspectral scanning systems attach simply to a microscope’s camera port and data acquisition is easy and fully automated. Visible and IR spectral ranges are available for measurement. The internal scanning system will allow hyperspectral microscopy to grow from a rare novelty into an affordable and widely accessible experimental technique.
References
[1] K.S. Banu, M. Lerma, S.U. Ahmed, and J.L. Gardea-Torresdey, Hyperspectral microscopy-applications of hyperspectral imaging techniques in different fields of science: a review of recent advances, Appl. Spectr. Rev. 59, 935 (2024). doi.org/10.1080/05704928.2023.2270035